I don’t drink soda.
Guy Fieri
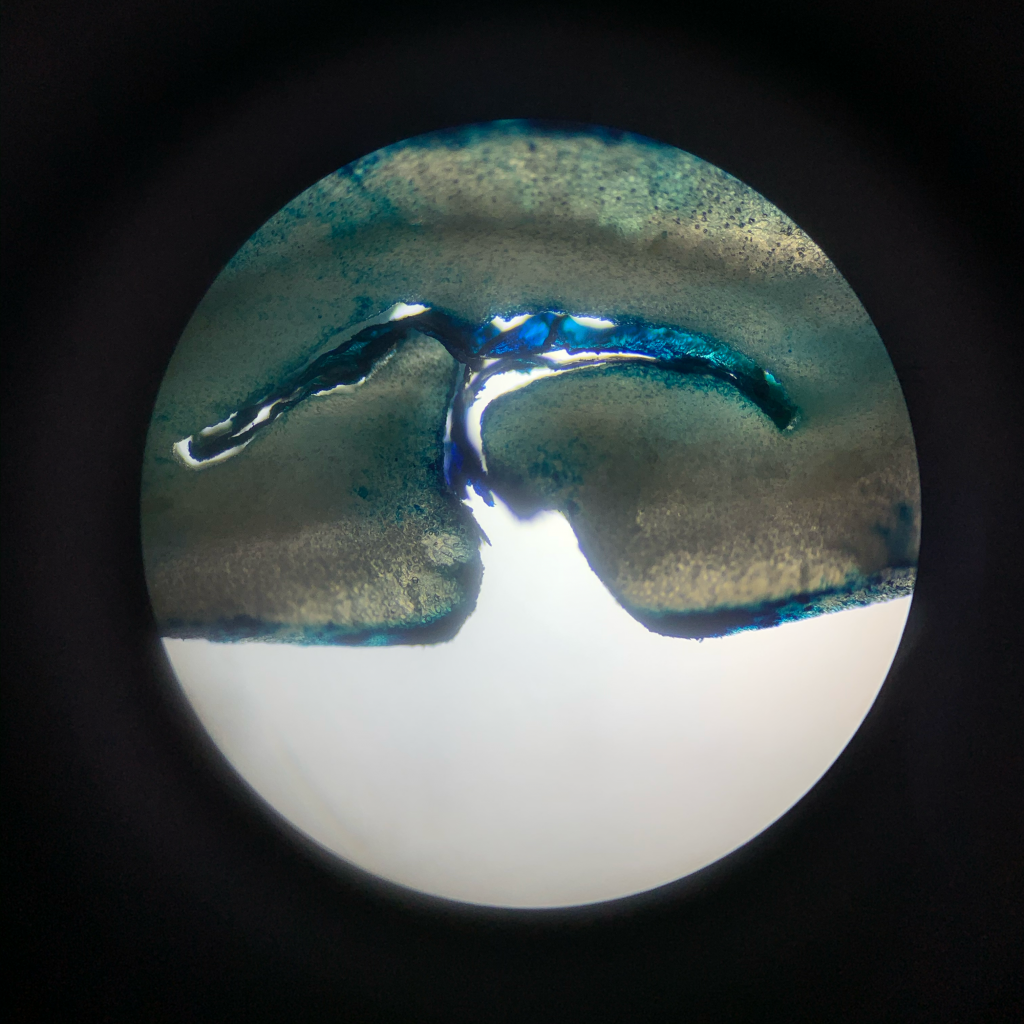
It was my fourth and final cupping of the day, and staring down at the mess of spent grounds and spilled coffee wrecking the table like seaweed at low tide or forgotten treasures unearthed in the snowmelt of spring, I dipped my spoon and set to work.
I had enough caffeine coursing through my veins by that point that either through transcendence or tachycardia, the odds were good I’d see god.
I’d taken 100g samples of green coffee and soaked them in eight different sodas (and one reference sample in brewing water) to rehydrate them, then dehydrated them mechanically to their starting moisture content, roasted and cupped them.
In the end, the coffees tasted like I expected them to—which was entirely the point.
Aida Batlle and I stood around a cupping table in El Salvador in 2017, tasting through samples from one of her recent projects. She’d been engaged to work with a wet mill in Mexico in the hopes of improving the robusta coffee her client processed there by employing some of the same processing techniques she uses at her micromill in Santa Ana.
In true Aida form, while some of the processes we tasted were relatively mundane (yeast inoculations, extended fermentations, dry fermentation followed by a soak, etc), some were more off-the-wall, such as the use of recycled pulp from Arabica in the tank of Robusta as well as the addition of “flavor crystals” to the fermenting coffee: freeze-dried cascara tea powder.
The idea was that maybe we could use some of the “good tasting” material from the Arabica as a bath bomb for the “rougher” tasting Canephora.
The theory relies on one of those simple truths of coffee that, while nearly universally accepted today, wasn’t exactly a commonly held belief for much of the last century: processing practices can improve upon the raw material.
Once upon a time in ancient history—as I was helping her prepare for her Re:Co lecture—I called Lucia Solis’ attention to a quote from Coffee Processing Technology by Michael Sivetz, a seminal technical manual first published in 1963: “it has been well established that coffee does not improve as processing time increases especially while the coffee contains large amounts of moisture” (p.96). Back then, it was believed that processing was a necessary but risky step: removing the skin of a coffee berry allowed for it to dry more rapidly, but also increased the risk of defect resulting from issues of hygiene or fermentation gone awry.
But we know better now (at least about that)—and the offer sheets of roasters and importers, and the novel processing techniques winning Cup of Excellence and fetching high prices at auction bear that understanding out.
Coffee is a hygroscopic sponge—readily and greedily absorbing flavors and aromas it comes into contact with, whether from spilled kerosene (my favorite “fault” from my Q course), storage in a bourbon barrel, or things it’s exposed to while waiting for the mucilage to fall off.
It’s a crouton in fermentation soup.
Compounds produced or liberated via fermentation—esters, aldehydes, ketones, and carboxylic acids—absorb into coffee seeds during fermentation/maceration, much in the same way that a sponge dropped into a bucket of water will absorb water.
Water creates a highway for molecular traffic to transfer between the material in the tank and the seed—both to and from the seed: from the areas with higher concentration of molecules to areas with lower concentration.
But not every molecule produced or present in the fermentation environment will shuttle into the seed: some compounds under normal conditions are simply unable to pass through the pores of a cell wall and remain excluded from the seed and wash away, degrade, recombine, or otherwise dissipate at the termination of the maceration.
That’s why a fermentation inoculated with pineapple won’t necessarily taste like pineapple—and why coffee soaked in Coca Cola won’t necessarily taste like soda.
I’ve long advocated for fermenting coffee under water. This seems counterintuitive to many buyers who note that dry-fermented coffees seem to exhibit more vibrant acidity and intensity, particularly when fresh.
By contrast, coffees fermented underwater tend to present with quieter acidity—but usually with greater uniformity and cup structure. I often prefer submerged fermentation because of process advantages: starting with a saturated seed and uniform moisture content means drying can be better regulated and controlled. This, in turn, can often mean a greater potential shelf life and resistance to fade.
But: coffees fermented underwater can also be also less dense. That’s because—in addition to kick-starting the seed for germination—soaking coffee under water will extract from the seed, even as some compounds are soaking into it.
Density, of course, is a prized attribute for roasters. For producers, density matters, too: if a coffee is less dense, it will take more individual seeds to fill a bag that is traded at a regulated weight of, say, 70kg.
It’s a game of yields: to produce the same amount of finished, exportable coffee, a producer will need more cherry. More cherry means higher costs and a smaller total harvest—and if not accounted for, less revenue and lower profits. Like outturns, density can have very real impacts on a producer’s economic security.
So the question bugged me: how long does it take water to penetrate and saturate a coffee seed? The answer, to my knowledge, doesn’t exist in the literature—and none of my colleagues knew—so I had to come up with a way to find out. The impacts on quality, shelf life, and producer economic security would be material if we could optimize and find the point at which coffee is saturated with coffee—meaning, the point at which instead of diffusing into a seed, water begins to extract material from it.
But if we wanted to to use this to our advantage—and use the capillary action of coffee to promote quality or influence a coffee’s character—how can we optimize the time of the water contact to allow compounds to pass into the seed but, critically, prevent substances from being diluted or extracted from the seed, lowering its density and flavor intensity?
Most of what we do in coffee, of course, has something to do with water: rainfall and irrigation and soil’s ability to hold water impact coffee trees; water used to sort, clean, and process coffee; water removed from coffee to dry it to stability; water buoying giant ships filled with coffee between continents; water removed through dehydrating non-enzymatic browning reactions when we roast; and then water added back to roasted, ground coffee to extract its solids during brewing.
When I first started working on this piece, it stemmed from conversations surrounding rehydration of coffee after I published a protocol for rehydrating green coffee, at scale, back in March of 2021.
How long it takes for fluids to enter a coffee seed, distribute, and homogenize, it turns out, was an unstated centerpiece in my experiments with rehydrating coffee. There, we wanted to essentially hit the same benchmark: have coffee in contact with water no longer than necessary: if we waited too long, we risked oxidation and mold growth.
But if I could optimize the time it would take under ambient temperatures and atmospheric pressures for coffee to reach the center of the seed, I realized it could also have profound impacts on processing by allowing us to ferment dry (to create intense, vibrant acidity) and then use water to carry fermentation metabolites into the seed, increase uniformity, and also homogenize our starting moisture content ahead of drying—all without sacrificing density.
So I needed to find a way to trace the movement of water into the seed.
I’d hoped to use some sort of dye or pigment—figuring I could soak coffee in the dye and pull out a sample every hour or so and take a slice with a scalpel then examine it under a microscope.

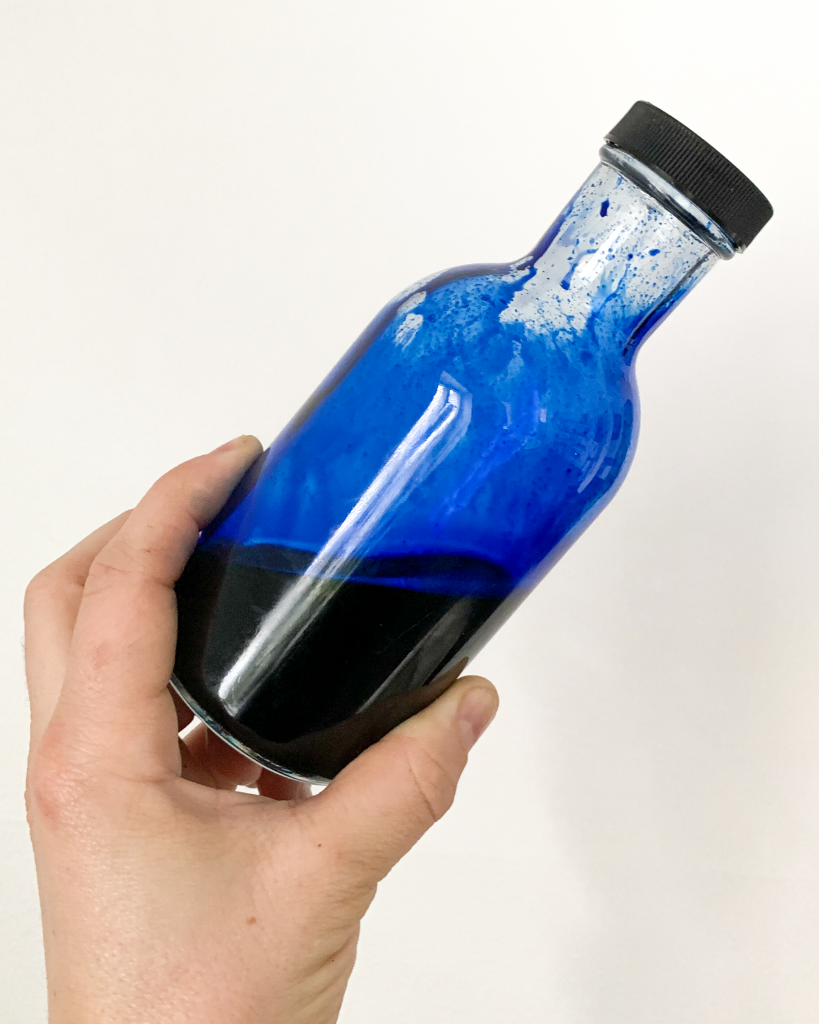
A 1% aqueous solution of methylene blue seemed to fit the bill: at around 14 angstrom by 9.5 angstrom in dimension and with a molecular mass of 319.9 g/mol, they could theoretically slide through the pore of a coffee cell. Further, methylene blue—which was the first synthetic antimalarial used in the late 19th and early 20th centuries—is highly soluble in water (43.6 g/L at 25ºc) and the most-commonly used dye in the textile industry as well as a commonly-used dye in studies of plant and animal tissues, so I figured the odds were good that it would work in this application.
MB works by staining living tissues containing acids blue—allowing us to clearly see the outline of cell walls and thus, I hoped, giving us a glimpse of water as it moved through the seed’s cellular matrix.
This was paper-napkin sketch exploration—not the stuff meant to be published and peer-reviewed—so I approached it pretty casually and didn’t control for the shape or density of coffee (in retrospect, I could have done a better job sorting and selecting for uniform geometry).
But regardless, we can clearly see the movement of the dye into and throughout the seed over the course of 10 hours:
[bonus: click to download a poster-size high-resolution image to print and display proudly in your lab]
From this, we can conclude that—at least for water and certain molecules, and under these conditions—it takes water about 10 hours to penetrate and saturate a coffee seed. And so the answer was known: adding a couple hours to account for the need to increase the time to account for permeability in the coffee pile during processing, we could theoretically redistribute molecules produced or made available during a fermentation into the seed in a soak of around 12 hours (with the rate of ingress slowing as the concentration matches between the inside of the coffee and outer environment, much like the slowing extraction over the course of an immersion brew).
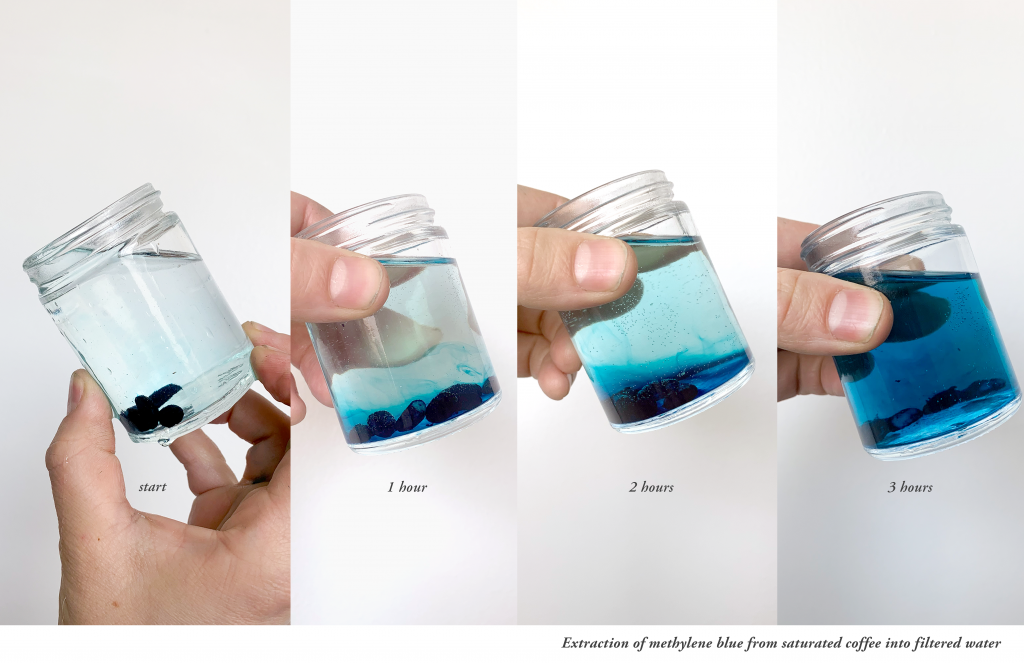
At the end of the 10 hours, I placed a few seeds in clean water—just to see what would happen if, for example, we had a second soak following fermentation, or if washing took a very long time—and within 3 hours, the color of the water was blue and the dye appeared to have mostly extracted from the seed.
But MB is a dye—what about other molecules?
For years, I’ve lived with the understanding—one I’ve heard shared by others in this space like Lucia—that sugar would not pass into a seed during fermentation or dry processing simply due to the size of the molecule. But there are other ways to make molecules shape shift and capable of fitting square pegs into round holes.
I became aware of a paper from Nottingham University ("Enhancing Robusta coffee aroma by modifying flavour precursors in the green coffee bean")
thanks to a tip from Samo Smrke after he read my piece on infused coffees. In it, the study’s authors describe soaking robusta in sugar solutions (of glucose, fructose, and sucrose, of varying concentrations) in an effort to improve the sensory quality of the coffee.
In the end, they were able to increase the concentration of sugar in a coffee seed, and one of the solutions they used—one with 15g fructose per 100g of water—outperformed the others, increasing the quality of robusta significantly enough that it could be blended up to 80% with Arabica “without significant aroma differences” from 100% Arabica (a Kenya AA).
Without spending any effort worrying about the roasting or extraction parameters used for this experiment, the results are still fascinating: they got sugar into a coffee seed.
But how?
Well—they used quite a bit of energy: “To accelerate the diffusion of sucrose, glucose and fructose into the coffee beans, pre-soaking was carried out at 2 bar pressure.” This was done for 30 minutes using water at 100ºC—far higher temperatures or pressures than you’d ever see in processing. And then:
A rotation of 1 rpm was used to create even distribution of the treatment solution. The process control (water treated Robusta) was significantly lower in sucrose, glucose and fructose content when compared with the non-treated Robusta. This is due to the nature of the treatment process as, sucrose, glucose and fructose are water soluble and can be leached out into the process water during the treatment.
(p. 9)
And even under those conditions, less sucrose penetrated the seed compared with glucose or fructose. As the authors explain: “Sucrose is a disaccharide with the molecular weight 342 g/mol and may penetrate the sample matrix less readily than monosaccharides such as glucose (180 g/mol) and fructose (180 g/mol).” [water has a molecular weight of 18 g/mol]
This is worth noting because sucrose, a non-reducing sugar, is present in about double the concentration in Arabica coffee versus Robusta (while glucose is roughly the same between the two species and robusta contains more fructose).
If you dive in a little deeper, you’ll notice that the initial concentration of sugar in dry Robusta (3.20g/100g) was higher than the sugar content of the treated samples of 3g/6g/9g/100g solutions and fell within the standard deviation for 12g/100g—meaning that, initially, the concentration of sucrose in the seed decreased as a result of the treatment and it required a high concentration differential between the solution and the coffee for the amount of sucrose to go into the seed in levels that exceeded the starting material.
This wasn’t the case for glucose or fructose—molecules about half the size of sucrose—which readily moved into the seed under these conditions.
Are we wrong about sugar’s ability to enter a coffee seed?
More pocket science—and a little math.
If you’re fermenting coffee in a 55 gallon drum (as is common to do), the total volume is 0.20m3. Let’s fill half of the tank with pulped coffee, half with fruit, and top off with water. How would that compare to the conditions in the Robusta study—In a real-world scenario, would concentrations of sugar be high enough to drive it into the seed?
Based on industry standard assumptions about the density of wet parchment coffee, if we filled our tank halfway, we’d be starting with about 73kg of pulped coffee. The bulk fill density of strawberries is roughly 602.23 kg/m3, meaning we’ll need to add 60kg of strawberries to fill the remainder of the barrel (that’s an imperial fuckton of strawberries, but bear with me here).
Knowing those two densities, we can make some assumptions and calculate about how much water we’d need to add to the tank to cover it to the top (since the mass we added to the tank would displace volume)—and we’d end up with 76.89L.
Ripe strawberries contain about 4.9g of sugar per 100g—divided about evenly between sucrose and fructose, or about 2.5g/100g of each.
But we added water: Suddenly, our concentration of sugars is diluted from 2.5g/100g to 1.09g/100g of strawberry-water surrounding the coffee.
This is below the lowest concentration of solutions used in the Robusta study, but is still higher than dry green coffee, which would have a starting concentration of 0.13g/100g for fructose and 0.36g/100g for glucose.
And of course, we haven’t yet factored in mucilage, which we’d need to first degrade to access the seed. Mucilage is about 4.1% reducing sugars (pretty close to strawberry) and 5% of the weight of a cherry—roughly 10% of the weight of the coffee in the tank. But mucilage is degraded in conventional fermentations (meaning those without adding citric acid or enzymes to expedite removal of the mucilage) as a byproduct of microbial activity; as the fermentation progresses, the amount of sugar decreases as the microbes consume it. Thus, by the time the mucilage is degraded enough to expose the seed, the amount of sugar in the tank will have fallen, since the microbes wouldn’t just selectively consume the sugars from the mucilage—they’d consume sugar from the strawberry-water, too.
A coffee cell is a rather “tight” structure, with just 7% pore volume with those pores having a diameter of, on average, about 10nm. Even with the study’s retort process—which, like during decaffeination processes using steam, enlarges the cell pores further and makes it easier to move molecules out of the seed—and much higher concentrations of sugar than the ones we’ve mathed here, the concentration of sugars never reached equilibrium between the coffee and the soak.
But in a coffee tank—even one loaded with an unreasonable amount of strawberries—we’re looking at significantly lower concentration of sugars, less heat (coffee fermentations don’t even come close to 100ºc), and less pressure (it’s conceivable that a pressurized fermentation tank could reach 2 bar but in most cases there is an airlock to prevent gas buildup and/or eruption).
As a result of this lower energy state, the coffee would be a more resistant to sugar absorption, and it’s unlikely that any significant amount of sugar would diffuse into the seed in a real-world scenario.
All that extra sugar does nothing other than feed microbes, who, in turn, turn it into smaller molecules (esters, aldehydes, ketones, carboxylic acids) that do enter the seed—coming and going like commuters with a monthly unlimited pass.
I wanted to know, though: how could I exaggerate and demonstrate this sort-of-complicated principle on a cupping table so that it’s easily understood and memorable?
I looked again at the concentrations of sugar used in the Robusta study.
Fuck, I realized. It’s soda.
For example: Coca-Cola, a nearly-universally recognized soda (one that I’ve seen everywhere in the world I’ve ever been) contains 10.6g of sugar per 100ml (in addition to water, caramel color, undisclosed natural flavors, phosphoric acid and caffeine), well within the range of sugar concentrations used in the study.
What better way to show the stubborn unwillingness of sugar to go into coffee than by soaking green coffee in soda?
(Plus: I thought it was hilarious)
I reached out to my Instagram followers, asking them what their favorite sodas were (I’m not a soda drinker). Based on the top results, I visited the Latin foods grocer in my neighborhood and stocked up:
I wanted to rehydrate coffee with a range of sodas and use buckshot to get an overall picture of what if any cup impacts these soda rehydrations would have.
Most of these sodas are sweetened with High Fructose Corn Syrup (HFCS), a combination of glucose and fructose in water (at a ratio of roughly 55% glucose to 45% fructose), but two (Mexican Coke and Mexican Sprite) were sweetened with sucrose (a disaccharide made of one molecule of glucose chemically bonded to one molecule of fructose).
The sugar content of the sodas are as follows:
- Jarritos Tamarindo (HFCS): 9.5g/100mL
- Mexican Sprite (Sucrose): 10.1g/100mL
- Mexican Coke (Sucrose): 10.3g/100mL
- Coca-Cola (HFCS): 10.6g/100mL
- Fanta Grape (HFCS): 10.9g/100mL
- Dr. Pepper (HFCS): 10.9g/100mL
- Sprite (HFCS): 11.5g/100mL
- Mountain Dew (HFCS): 13g/100mL
Anywhere you see HFCS, you can infer that for the total concentration of sugar listed, about half is fructose and half is glucose, while any sweetened with sucrose the total concentration refers solely to that sugar.
All contain some sort of flavoring, coloring, and acids.
I rehydrated 100g samples of a fairly neutral and clean coffee—a washed Caturra from Guatemala grown at around 1350 masl—in pint containers filled with one of the sodas. As a reference, I also rehydrated a sample with brewing water as well as a final wildcard—which I labeled “jungle juice”—a mixture of all of the sodas sloshed together.

In the Robusta experiment, the scientists exposed coffee to sugar syrups under retort conditions for just 30 minutes. In ambient conditions (approximately 22º in my lab), I’d need to lean on the understanding of diffusion into a seed that I’d developed with my study of methylene blue and allowed the coffee to soak in sodas for 10 hours (the rule of thumb is that rate of reactions doubles for every 10ºC increase in temperature—not even including considerations for pressure or materials weakening—so we’d be looking at 64 hours of soak if we played it straight to match the accelerated rates at 100ºC. Maybe next time)
After 10 hours elapsed, I drained the coffees, placed them on clean mesh trays in a dehydrator set to 30ºC and cycled it in day/night cycles (12 hours on, 12 hours off), storing the coffee during the cycles of rest in sealed containers to allow moisture to redistribute within each sample. When the coffee reached its initial moisture content, I roasted all 10 rehydrated samples and a reference sample of the original untreated coffee using the same profile on an IKAWA sample roaster, allowed the coffee to rest for two days, and then cupped them blind.
At the end of this effort, the results were rather unimpressive: the rehydrated samples all outperformed the untreated coffee and yet were nearly indistinguishable from each other.
I narrowly preferred the Coca-Cola and Dr. Pepper rehydrated coffees, finding them to be somewhat more tangy and have a slightly more intense acidity compared to the water rehydrated sample—but if I’d taken the time to do a triangulation I’m not convinced I could have detected a difference. None of them tasted anything like the sodas they bathed in, with the exception of one—Fanta Grape—which had an ever-so-slight concord grape note to it (which tasted utterly artificial and didn’t integrate into the cup).
And finally: the sucrose-sweetened Mexican Coke and Mexican Sprite performed indistinguishably from their HFCS-sweetened counterparts.
This was, of course, the null hypothesis—as well as what I expected to happen.
After I conducted this experiment, I reached out to the producer whose coffee I used and shared my findings. I’ve bought coffee from their farm since 2017, and as their largest single contract each year, we work together each year to come up with a plan for how the coffee will be picked, sorted and processed. We work to optimize the process to ensure that the coffee cups as well as possible and resists fade for a full calendar year and adjust the price paid accordingly.
For 2022, rather than fermenting under water—which we’d done in years past and which is the standard process at their wet mill—we first fermented the coffee dry (with a selected yeast added) and allowed the fermentation to progress for 22 hours in clean, open-air sealed tile tanks under shade. After 22 hours, the fermentation was “complete”—the mucilage was falling off, and the tank smelled tropical and ripe. We could have, at this point, moved the coffee through the channels to wash it, as is typically done at this point.
Instead, we added clean water to the tank, just covering the top of the coffee. The coffee was manually mixed in the tank with clean paddles, shedding its mucilage as it was turned, allowing the water to permeate the pile more readily and rapidly. And then it sat: a crouton in fermentation soup, absorbing everything we’d produced during the fermentation—and, we hoped, avoiding egress of any compounds that might reduce the density of the coffee and intensity of the cup.
We allowed the coffee to soak and absorb the water for 12 hours, then moved it without washing to raised beds to drip for a day before drying it mechanically at low temperatures with simulated day/night cycles and periods for rest and moisture stabilization throughout.
When the coffee arrived to the U.S. six months later—late, as is the nature of global logistics in 2022—it didn’t show any degree of fade, cupped a half point higher than its reference, and recorded higher bulk fill density than coffees picked and processed conventionally from the neighboring parcels.
And if we’d soaked it in Coca-Cola anywhere along the way—you’d never know.
Tags: coffee green coffee moisture processing
Prosecco is the great missing of this experiment… next time :sigh:
*chef’s kiss*
Awesome read. Really amazing effort. I am wondering though: the Meth blue experiment I agree is a great visual aid, but if you plan to implement your findings to an entire coffee lot, why not set up multiple small scale tests and remove from soak at different time points. This way you get to actually cup the real result, which in my head would be much more useful for future choices of processing in the next and next next harvest. Perhaps you already do this, but wanted to share an entertaining post (which it was)?
I have used both wine (rosé) and beer (porter) in a similar experiment protocol with good results. They don’t have high concentrations of saccharides but of course a lot of desirable fermentation compounds. The beans’ inherent flavor was overwhelmed though..
It is really interesting to see that the soda coffee tasted good. We have seen a good number of interesting yeast related processing methods, and even some with extracts and fruits involved. I wonder if juice rehydration will become the next new thing
Yes, I’ve been working with yeast for years and have enjoyed the predictability of the outcomes.
Found this link on Lucia’s discord group. You write, “the rehydrated samples all outperformed the untreated coffee and yet were nearly indistinguishable from each other.” I wonder how the rehydrated coffee samples outperformed the untreated coffee. I think you proved that the coffee did not absorb the sugar from the soda because the coffee rehydrated with just water was nearly indistinguishable in cup profile. I assume that means the rehydrated coffees were not “sweeter” than the untreated coffee. Could you describe the difference you perceived on the cupping table between the control (not rehydrated) and rehydrated coffee? And thank you for writing such thought-provoking content.
Hey there, absolutely. It matched well my experiences with rehydrating coffee — I recommend reading those three posts (linked from the homepage) for more info but essentially it presented with greater aroma, intensity, body, and a more “viscous” acidity. Tangy, creamy. All of the rehydrated samples were sweeter—but the soda rehydrated were not any sweeter than the coffee rehydrated with water. The reference un-hydrated coffee was, by comparison, quiet.
I tried to repeat this experiment, but added a batch with 2 bars of pressure on the beans soaking in soda.
Also let it soak for 100-120 hours in the fridge.
I’m pretty certain there was more sucrose in the dried beans, both with and without pressure. Both batches took on some of the soda flavor, but the pressurised absorbed much more and was cloyingly sweet.
Not at all tasty, but the main issue is that around 180-190C the sucrose starts to burn and emit smoke. I have experienced this before, with beans soaked in GoodBelly Blueberry. I guess some of the sucrose survives the roast, but a substantial amount turns into something reminding of the burnt edges on crispbread. Follow the link for pictures (auto-translated, sorry).
Awesome read. Really amazing effort. I am wondering though: the Meth blue experiment I agree is a great visual aid, but if you plan to implement your findings to an entire coffee lot, why not set up multiple small scale tests and remove from soak at different time points. This way you get to actually cup the real result, which in my head would be much more useful for future choices of processing in the next and next next harvest. Perhaps you already do this, but wanted to share an entertaining post (which it was)?